Magmatic flow
The fabric in some rock bodies looks different and has different properties in different places in the body. Obviously, this inhomogeneity, or heterogeneity, depends on the scale of observation. For example, an aphyric, holocrystalline basalt appears homogeneous on the scale of a hand sample, but, on a microscopic scale, a plagioclase in one place in the field of view is certainly not the same as a pyroxene in another; on this scale the basalt is inhomogeneous.In extrusive rocks, evidence of flow is most clearly shown as flow lines in glassy volcanic rocks; they are commonly contorted and folded during the flow and may be delineated by concentrations of crystallites or microlites (Fig.1). Magmatic flow involves change of shape of magma, with suficient melt for the crystals to rotate passively into alignment without them deforming internally. However, magmatic flow microstructures are generally less obvious in coarse-grained intrusive rocks, they may be spectacular; for example, the plagioclase crystals in some gabbros and cumulates have strong preferred orientation. This is probably mainly caused by flow currents in the magma chamber and/or rotation during compaction. The microstructural evidence for magmatic flow includes:
(1) parallel or subparallel alignment of elongate euhedral crystals, commonly feldspar, hornblende, or olivine, that are not internally (plastically) deformed; (2) imbrication of elongate euhedral crystals that are not internally deformed or of fragments of phenocrysts that have undergone boudinage; (3) contorted and folded flow lines (commonly caused by concentrations of minute crystals and/or devitrification products) in glassy volcanic rocks (Fig.1); (4) elongation of gas bubbles. (5) elongation of microgranitoid enclaves; (6) layers (schlieren) of crystals of mainly mafic minerals formed by sorting during magmatic flow, in absence of plastic deformation of the minerals involved.
.png)
Fig.1: Sketch showing microstructural features ideally developed around a rotating phenocryst in a relatively homogeneous groundmass flowing by simple shear. Compression of the flow layers occurs at X, expansion occurs at Y, and deflection around the phenocryst occurs at Z. the small red arrow show the direction of closure of the microfold and the black arrow show the sense of rotation of the phenocryst. Modified from Vernon (2008).
Magmatic foliation
Magmatic foliation can be expressed or defined in different ways:1. In some volcaniclastic deposits, layering is called bedding, or stratification. Layering is expressed by planar contrasts and heterogeneities in texture (grain and pore size and/or shape) or mineral modal proportions. Various names have been applied to layering that is defined by contrasts in modal proportions of minerals, but the nongenetic term compositional layering is appropriate.
2. Another expression of foliation is inequant mineral grains, whose longest dimensions are preferentially oriented within parallel planes. Oriented platy micas are a common expression of foliation. Tabular grains, such as feldspars, can have their two longest dimensions preferentially oriented in planar fashion. Planar preferred orientation of tabular feldspars in phaneritic rocks, or igneous lamination, is the trachytic texture.
3. Another expression of foliation in granitic rocks is schlieren (singular, schliere). These are oriented wispy, diffuse concentrations of mafic minerals in a more leucocratic matrix that may be planar disks, planar-linear blades, or linear pencil-like shapes.
Origin of magmatic flow and oriented textures
Magmas convecting in staging chambers within the crust, moving through overlying conduits, and flowing onto the surface of the Earth create anisotropic flow fabrics. Magma flow occurs by movement of viscous melt between rigid suspended crystals. Flow velocity is slowest along fixed boundaries of a moving magma body but can increase inward, creating a gradient in flow velocity. The pattern of velocity gradients and how internal flow markers interact with it result in different forms of anisotropic fabric. Suspended rigid crystals, volatile-fluid bubbles and distinctive mineral aggregates embedded in magma can serve as flow markers.Consider, first, flow orientation of inequant grains and grain aggregates. If inequant grains of columnar shape are caught in flowing magma that has a velocity gradient, the columns rotate into a stable orientation parallel to the velocity vectors, as logs do in a river, forming a lineated fabric (Fig.2a). In the same velocity-gradient flow regime, tabular feldspars rotate into a stable orientation that imparts both foliation (igneous lamination) and lineation to the magmatic body (Fig.2b). Consider, second, the origin of flow layering. Ridge and valley profiles of velocity in flowing magma can also translate grains of almost any shape toward the higher velocity region. In three dimensions, if the velocity gradients are nonuniform in a planar sense (Fig.2c), then planar flow layering, expressed by layers of greater and lesser concentrations of crystals, can develop. Extruded, viscous magmas commonly have a flow layering that is expressed by varying planar concentrations or sizes of crystals or of vesicles that may have originated in this manner.
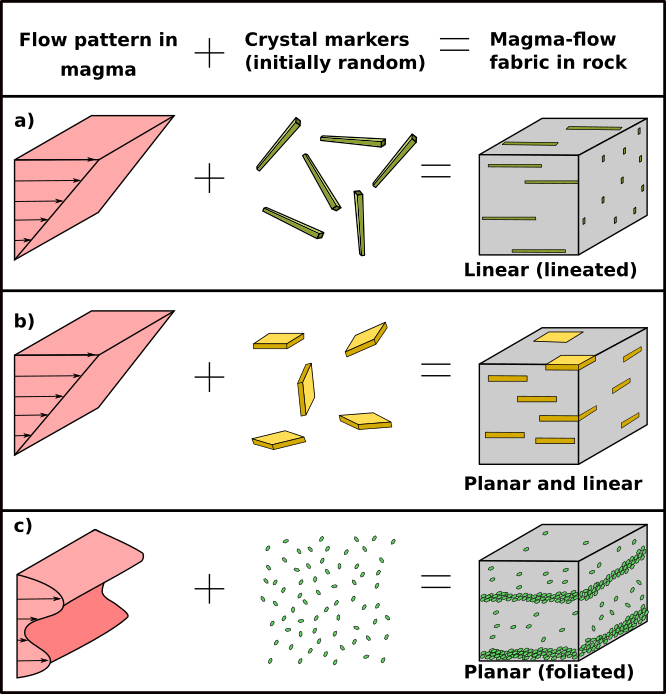
Fig.1: Sketch showing microstructural features ideally developed around a rotating phenocryst in a relatively homogeneous groundmass flowing by simple shear. Compression of the flow layers occurs at X, expansion occurs at Y, and deflection around the phenocryst occurs at Z. the small red arrow show the direction of closure of the microfold and the black arrow show the sense of rotation of the phenocryst. Modified from Vernon (2008).
Bibliography
• Cox et al. (1979): The Interpretation of Igneous Rocks, George Allen and Unwin, London.
• Howie, R. A., Zussman, J., & Deer, W. (1992). An introduction to the rock-forming minerals (p. 696). Longman.
• Le Maitre, R. W., Streckeisen, A., Zanettin, B., Le Bas, M. J., Bonin, B., Bateman, P., & Lameyre, J. (2002). Igneous rocks. A classification and glossary of terms, 2. Cambridge University Press.
• Middlemost, E. A. (1986). Magmas and magmatic rocks: an introduction to igneous petrology.
• Shelley, D. (1993). Igneous and metamorphic rocks under the microscope: classification, textures, microstructures and mineral preferred-orientations.
• Vernon, R. H. & Clarke, G. L. (2008): Principles of Metamorphic Petrology. Cambridge University Press.