Volcanic Rocks
extrusive rock, any rock derived from magma (molten silicate material) that was poured out or ejected at the Earth’s surface. By contrast, intrusive rocks are formed from magma that was forced into older rocks at depth within the Earth’s crust; the molten material then slowly solidifies below the Earth’s surface, where it may later be exposed through erosion. Extrusive rocks are usually distinguished from intrusive rocks on the basis of their texture and mineral composition.The main factor that determines the texture of an igneous rock is the cooling rate (dT/dt).
Other factors involved are:
• The diffusion rate - the rate at which atoms or molecules can move (diffuse) through th liquid.
• The rate of nucleation of new crystals - the rate at which enough of the chemical constituents of a crystal can come together in one place without dissolving.
• The rate of growth of crystals - the rate at which new constituents can arrive at the surface of the growing crystal. This depends largely on the diffusion rate of the molecules of concern. In order for a crystal to form in a magma enough of the chemical constituents that will make up the crystal must be at the same place at the same time to form a nucleus of the crystal.
Once a nucleus forms, the chemical constituents must diffuse through the liquid to arrive at the surface of the growing crystal. The crystal can then grow until it runs into other crystals or the supply of chemical constituents is cut off.
All of these rates are strongly dependent on the temperature of the system. First, nucleation and growth cannot occur until temperatures are below the temperature at which equilibrium crystallization begins. Shown below are hypothetical nucleation and growth rate curves based on experiments in simple systems. Note that the rate of crystal growth and nucleation depends on how long the magma resides at a specified degree of undercooling (DT = Tm - T), and thus the rate at which temperature is lowered below the the crystallization temperature.
.jpg)
At larger degrees of undercooling, the nucleation rate will be high and the growth rate also high. This will result in many crystals all growing rapidly, but because there are so many crystals, they will run into each other before they have time to grow and the resulting texture will be a fine grained texture. If the size of the grains are so small that crystals cannot be distinguished with a hand lens, the texture is said to be aphanitic.
At high degrees of undercooling, both the growth rate and nucleation rate will be low. Thus few crystals will form and they will not grow to any large size. The resulting texture will be glassy, with a few tiny crystals called microlites. A completely glassy texture is called holohyaline texture.
Two stages of cooling, i.e. slow cooling to grow a few large crystals, followed by rapid cooling to grow many smaller crystals could result in a porphyritic texture, a texture with two or more distinct sizes of grains. Single stage cooling can also produce a porphyritic texture. In a porphyritic texture, the larger grains are called phenocrysts and the material surrounding the the phenocrysts is called groundmass or matrix.
Another aspect of texture, particularly in medium to coarse grained rocks is referred to as fabric. Fabric refers to the mutual relationship between the grains. Three types of fabric are commonly referred to:
1. If most of the grains are euhedral - that is they are bounded by well-formed crystal faces. The fabric is said to be idomorphic granular.
2. If most of the grains are subhedral - that is they bounded by only a few well-formed crystal faces, the fabric is said to be hypidiomorphic granular.
3. If most of the grains are anhedral - that is they are generally not bounded by crystal faces, the fabric is said to be allotriomorphic granular.
Silicate Melt Structures
The structure of a silicate melt, like that of a silicate mineral, is formed by a 3D network of linked silica tetrahedra. In silicate melts, however, the structure is more disordered, is flexible and can rearrange itself through the random motions of atoms and molecules. The network in the melt contains many irregular rings and chains..jpg)
Structure of a silicate melt
Atoms in the structure play the same roles as in silicate minerals. There are network formers (Si), bridging oxygens (O0) and non-bridging oxygens (O-) making up the tetrahedra network, free oxygens (O2-) that are not part of tetrahedra, and network modifiers that support and change the shape of the silicate network.
To construct the silicate network we must add silica tetrahedra to the framework, turning non-bridging oxygens into bridging oxygens. This process of building a larger molecule by the repeated addition of smaller molecules is known as polymerisation and the network itself is a polymer.
A more polymerised magma will have a higher viscosity than a less polymerised magma. This is because the silicate network, although flexible, consists of strong directional covalent bonds that resist deformation. Viscosity is simply a measure of the resistance of a liquid to flow, and flow requires the structure to deform and move. The more polymerised the magma the less easily it can deform and the higher its viscosity.
Density, to a lesser extent, is also dependent on the degree of polymerisation. Magmas with large polymers tend to be less dense since their silicate network contains open rings. Magmas with a low degree of polymerisation are more compact and thus denser. The abundance of metal cations is also important in controlling the density of a magma.
The Effects of Temperature and Pressure
Increasing temperature results in depolymerisation of the silicate network of a magma because the increased kinetic energy of atoms makes it easier for bonds to be broken. Since the magma becomes more depolymerised it, therefore, becomes less viscous (density usually still decreases, however, due to expansion of the melt). Decreasing temperature causes an increase in polymerisation for the opposite reason and increases the viscosity.
Increasing pressure results in polymerisation of the silicate network of a magma because the structure is compressed and new bonds between tetrahedra form. Increasing pressure causes increases in viscosity and density of the magma.
Silica Content
Increases in the SiO2 content of a magma produces large increases in the degree of polymerisation since there are more SiO44- tetrahedra available to construct the network. Silica-rich (acid) magmas are, therefore, much more viscous and have a lower density than silica-poor (basic) magmas. The addition of any network former will cause an increase in polymerisation.
Volatiles
Volatiles are gaseous substances such as CO2 and H2O. They are very important in the behaviour of magmas during eruption, they change the melting point of magmas and they change their physical properties. Water and CO2, however, behave differently when incorporated into magmas.
Water
In the below diagram is represented the reaction mechanism by which water dissolves in a silicate magma. The hydrogen of the H2O molecule reacts with a bridging oxygen of the silicate network to form a hydroxyl (OH) molecule which is hydrogen bonded to a bridging oxygen. The reaction of the H2O molecule causes depolymerisation. Adding water to a silicate melt will, therefore, decrease its viscosity. The viscosity of polymerised melts, such as acidic melts, decreases more significantly with water content than less polymerised (basic) melts.
.jpg)
Reaction mechanism by which water dissolves in a silicate magma.
The degree of polymerisation also controls the amount of water that can be dissolved in a magma. Polymerised magmas (acidic) can dissolve large amounts of water since they have more bridging oxygens. The solubility of water in magmas, therefore, decreases with SiO2 content due to the decrease in polymerisation (i.e. acid magmas can contain more dissolved water than basic magmas).
With decreasing pressure the solubility of water in a magma decreases because of the decrease in polymerisation. The explosive volcanism of acid magmas is a result of this behaviour since as they rise through a conduit the pressure decreases until the water can no longer be dissolved in the magma and exsolves as water vapour. The large expansion of the vapour causes the explosive nature of the eruption.
Carbon Dioxide
Carbon dioxide (CO2) dissolves in a magma by reacting with a non-bridging oxygen of the silicate network to form a carbonate molecule (CO32-). The removal of the non-bridging oxygen causes polymerisation of the network as shown and thus increases in viscosity. Because CO2 solubility is dependent on non-bridging oxygens it is higher in depolymerised magmas. The CO2 content of basic magmas is, therefore, higher than acidic magmas.
.jpg)
Reaction mechanism by which Carbon Dioxide dissolves in a silicate magma.
A complication is in the presence of Al3+ as a network former (i.e. in the tetrahedra) since the carbonate ion formed by the reaction of CO2 forms a complex with a divalent cation which can no longer balance the charge of the Al in the network. In the case of Al-rich magmas CO2 can cause depolymerisation.
Magmatic series
The first major division is based on the alkali (soda + potash) and silica contents, which yield two groups, the subalkaline and alkaline rocks.Subalkaline:
Chemically the subalkaline rocks are saturated with respect to silica; consequently, they have normative minerals such as orthopyroxene and quartz but lack nepheline and olivine (in the presence of quartz). This chemical property also is reflected in the mode of the basic members that have two pyroxenes, orthopyroxene and augite. Plagioclase is common in phenocrysts, but it can also occur in holocrystalline rocks in the microcrystalline matrix along with the pyroxenes and an iron–titanium oxide phase. In addition to the differences in iron content between the tholeiitic and calc-alkalic series, the latter has a higher alumina content (16 to 20 percent), and the range in silica content is larger (48 to 75 percent compared to 45 to 63 percent for the former). Hornblende and biotite phenocrysts are common in the calc-alkalic andesites and dacites but are lacking in the tholeiites except as alteration products.
Tholeiitic and Calc-alkaline
Rocks in the tholeiitic magma series are classified as and are distinguished from rocks in the calc-alkaline magma series by the redox state of the magma they crystallized from (tholeiitic magmas are reduced; calc-alkaline magmas are oxidized). When the parent magmas of basalts crystallize, they preferentially crystallize the more magnesium-rich and iron-poor forms of the silicate minerals olivine and pyroxene, causing the iron content of tholeiitic magmas to increase as the melt is depleted of iron-poor crystals. However, a calc-alkaline magma is oxidized enough to precipitate significant amounts of the iron oxide magnetite, causing the iron content of the magma to remain more steady as it cools than with a tholeiitic magma.
The difference between these two magma series can be seen on an AFM diagram, a ternary diagram showing the relative proportions of the oxides Na2O + K2O (A), FeO + Fe2O3 (F), and MgO (M).
.jpg)
AFM diagram showing "typical" areas for various extents of evolution from primitive magma types. Tholeites go through a Ferro-Basalt stage before continuing towards Rhyolite.
As magmas cool, they precipitate out significantly more iron and magnesium than alkali, causing the magmas to move towards the alkali corner as they cool. In the tholeiitic magma, as it cools and preferentially produces magnesium-rich crystals, the magnesium content of the magma plummets, causing the magma to move away from the magnesium corner until it runs low on magnesium and simply moves towards the alkali corner as it loses iron and any remaining magnesium. With the calc-alkaline series, however, the precipitation of magnetite causes the iron-magnesium ratio to remain relatively constant, so the magma moves in a straight line towards the alkali corner on the AFM diagram.
Alkaline Series
The alkaline rocks frequently occur on oceanic islands (usually formed during the late stages of magma consolidation after tholeiitic eruptions) and in continental rifts (extensive fractures). Based on the relative proportions of soda and potash, the alkaline series is subdivided into the sodic and potassic series. The alkaline rocks typically are chemically undersaturated with respect to silica; hence they lack normative orthopyroxene and quartz but have normative nepheline. Microscopic examination of the alkali olivine basalts usually reveals phenocrysts with an abundance of olivine, one pyroxene (augite, which is usually titanium-rich), and plagioclase. Nepheline may be seen in the matrix.
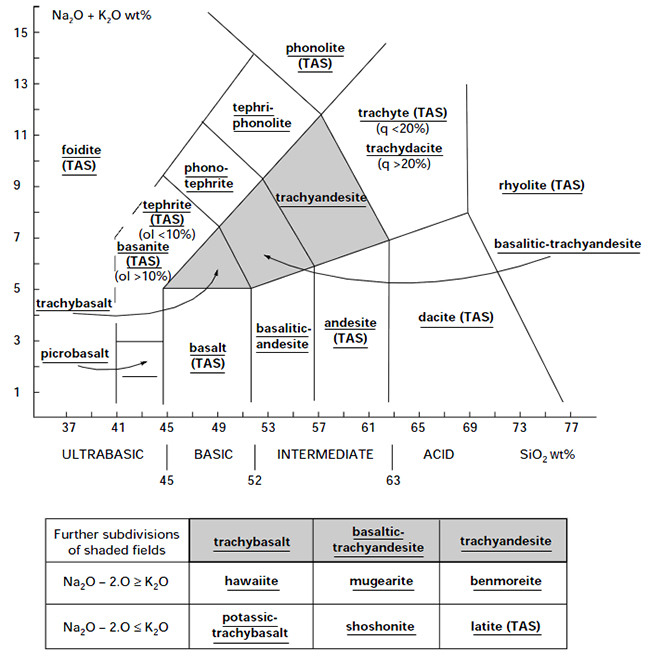
Chemical classification and nomenclature of fine-grained crystalline rocks using the total alkali silica (TAS) diagram (after Le Bas et al., 1986). Rocks falling on the shaded areas may be further subdivided as shown in the table underneath the diagram. The line drawn between the foidite field and the basanitetephrite field is dashed to indicate that further criteria must be used to separate these types. Abbreviations: q = normative quartz; ol = normative olivine. From IUGS recommendations